Education
- B.E., Engineering Physics, 2005, Tsinghua University
- M.E., Engineering Physics, 2007, Tsinghua University
- Ph.D., Mechanical and Aerospace Engineering, 2013, Princeton University
Background
Prof. Wenting Sun received his B.E. and M.E. degrees from Tsinghua University, Beijing in 2005 and 2007, respectively, and his Ph.D. degree from Princeton University in 2013. He joined Georgia Tech in July 2013. Dr. Sun’s research spans on combustion simulation, combustion kinetics, and plasma/ozone assisted combustion. He develops new numerical algorithms to accelerate large scale CFD simulation using predictive kinetic models. His work on plasma/ozone assisted combustion is to induce plasma generated species into combustion system to enable combustion at extreme conditions. Dr. Sun has developed a high pressure shock tube with unique capability allowing investigation of combustion kinetics for future power generation systems.
Research
- Dr. Sun’s research spans on combustion simulation, combustion kinetics of conventional and alternative fuels, and new combustion technologies to enhance combustion process. Key to his research is developing new numerical algorithms to accelerate large scale CFD simulation using predictive kinetic models. His work on plasma/ozone assisted combustion is to induce plasma generated species into combustion system to enable combustion at extreme conditions, such as those in supersonic combustion Ramjet engines hypersonic propulsion. Dr. Sun has developed a high pressure shock tube with unique capability allowing investigation of combustion kinetics at supercritical carbon dioxide conditions for the next generation of power generation systems. The new supercritical carbon dioxide oxy-combustion power generation system features high efficiency and almost 100% carbon capture, which will change the landscape of power generation. He also developed a novel super rapid combustion machine to study fundamental turbulence/autoignition interaction to improve combustion models.
(1) Kinetic Model Reduction and Dynamic Adaptive Kinetics for Turbulent Combustion
High-fidelity simulation of combustion systems is a critical element for combustor and engine design. However, to model a combustion system, a chemical kinetic model including hundreds, even thousands, of species and reactions are needed to describe the fuel oxidation and heat release process. For each species in the kinetic model, one ordinary differential equation needs to be solved. The complicated kinetic model makes high-fidelity simulation very challenging and even prohibited because of the limitation of the needed computation power. For example, for large-scale simulations such as LES or DNS, using a predictive detailed kinetic model is prohibited. Thus, there exists a gap between the development of a detailed, predictive combustion kinetic model and high-fidelity large-scale simulation. We work on developing new algorithms to enable and accelerate large scale numerical simulations on combustion with predictive kinetic models. Recently, we developed a new algorithm GPS (Global Pathway Selection) methond for kinetic model reduction to decrease the number of species in the detailed kinetic model efficiently from hundreds/thousands to tens through the analysis of element flux. Therefore, the reduced predictive kinetic models can be employed by LES or DNS for high-fidelity simulation. This algorithm constructs element flux graphs for considered elements, for example, C, O, and H for hydrocarbon combustion systems. Based on the constructed element flux graphs, important species which transfer significant element flux can be selected in the kinetic model. The global pathways for selected species can be identified by searching the shortest paths with the constructed element flux graphs. In this way, a reduced kinetic model can be constructed for use in complex CFD simulation. For given accuracy, the smaller the number of species needed in the reduced model, the more efficient the reduced kinetic model is, with the added benefit of considerably shorter simulation time. The GPS software and source code can be download here.
To further reduce the computation time of large-scale high-fidelity simulation, we developed a new numerical framework for DNS of turbulent combustion. The principle behind this multidisciplinary work is that different regions in the computation domain have different thermodynamic states; so only a small portion of species in the kinetic model needed to be calculated in the simulation. Therefore, different regions at different times can employ different reduced kinetic models generated on-the-fly with a much smaller number of species to further accelerate the simulation. The new framework was demonstrated using a canonical turbulent premixed flame employing a real jet fuel kinetic model (see plots blow). With high accuracy, the new numerical framework provides a significant speed-up of computation and the total CPU time is reduced by a factor of approximately 20. This new numerical framework enabled DNS with predictive kinetic models with good accuracy and parallel scalability.
The new regime-independent framework for 3D DNS of turbulent combustion with detailed kinetics is developed by incorporating on-the-fly adaptive kinetics (OAK), correlated transport (CoTran) techniques, and an efficient point-implicit ODE solver (ODEPIM) into a conventional DNS platform. All three methods are modified and optimized to adapt to 3D turbulent combustion and parallel high performance computing (HPC). A canonical turbulent premixed flame configuration corresponding to the thin reaction-zone regime is considered, where an initially planar premixed flame front interacts with a decaying isotropic turbulence. The computational domain consists of a cube with length 0.015 m. With the new numerical frame work, calculation of chemistry can be accelerated 46 times, calculation of transport can be accelerated 72 times, and overall acceleration of calculation is 20 times. See our publication here.
With the new capability enabled by the above-mentioned new numerical frame work, we further investigated the sensitivity of DNS predictions to chemical kinetic models. DNS of a canonical temporally evolving turbulent non-premixed flame was conducted using two different kinetic models. This simulation would not have been possible without the newly developed numerical framework discussed above. It was found that at laminar conditions, the two kinetic models provided very close predictions on combustion properties such as autoignition delays, flame speeds, and extinction strain rates. However, at turbulent conditions, different predictions were observed. The temperature predicted by these two kinetic models can vary by as much as 100 K. Detailed systematic analysis revealed that the sensitivity to the chemical kinetic models was magnified by the effects of unsteadiness and turbulence. This study has resolved an important question faced by the combustion community for a long time, that a different selection of kinetic models affects the prediction of DNS of turbulent combustion even though the kinetic models behave similarly at laminar conditions. See our related publication here.
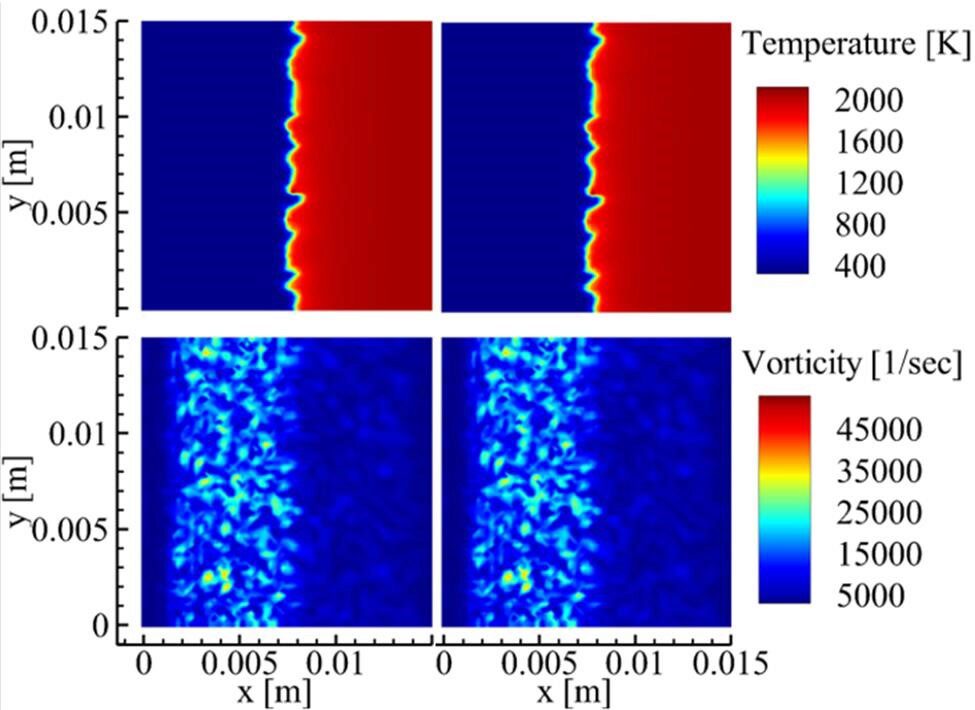
Temperature (upper) and vorticity (lower) at the center plane (z = 0.75 cm) using conventional DNS (left) and proposed framework (right)
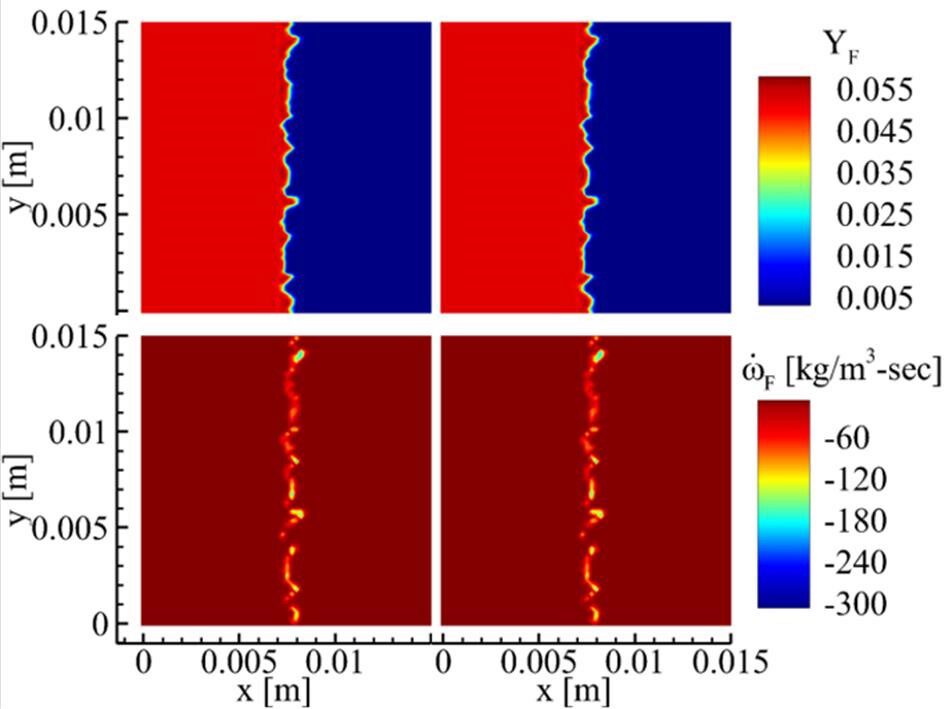
Mass fraction (upper) and reaction rate (lower) of fuel at the center plane (z = 0.75 cm) from conventional DNS (left) and proposed framework (right)
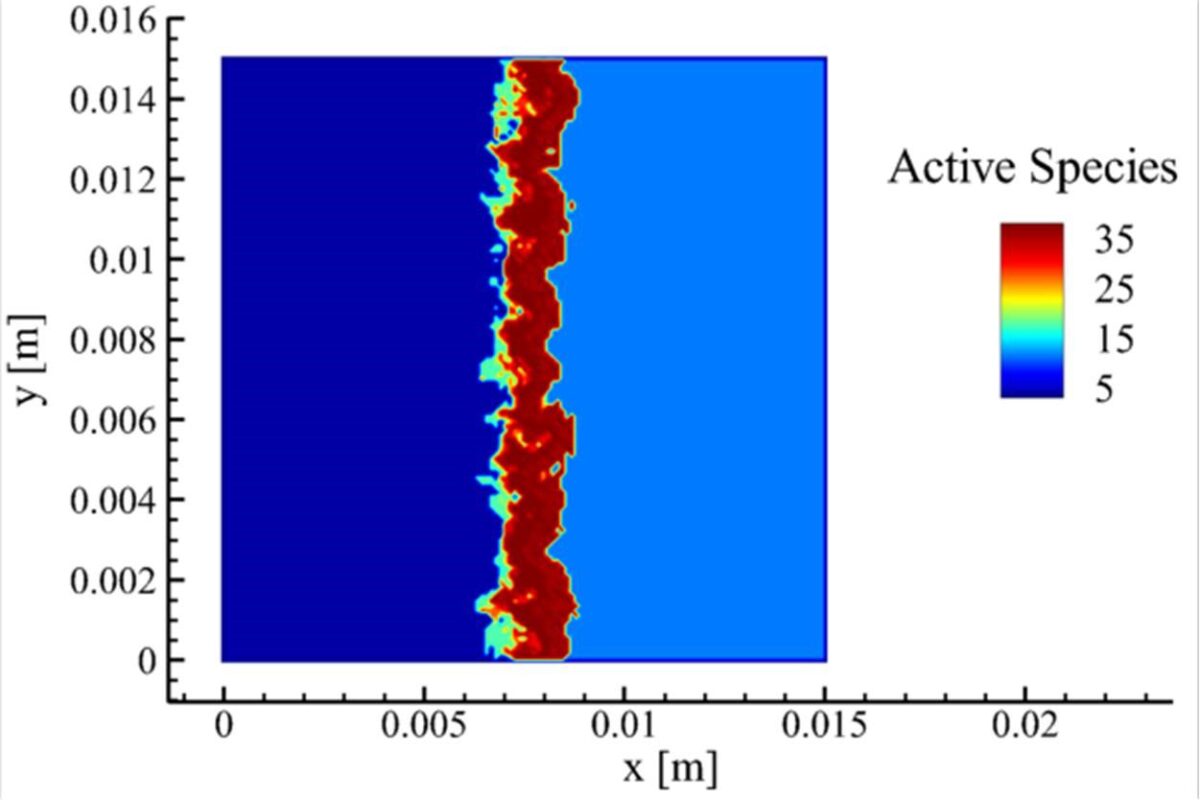
spatial distribution of the number of active species at the center plane (z = 0.75 cm)
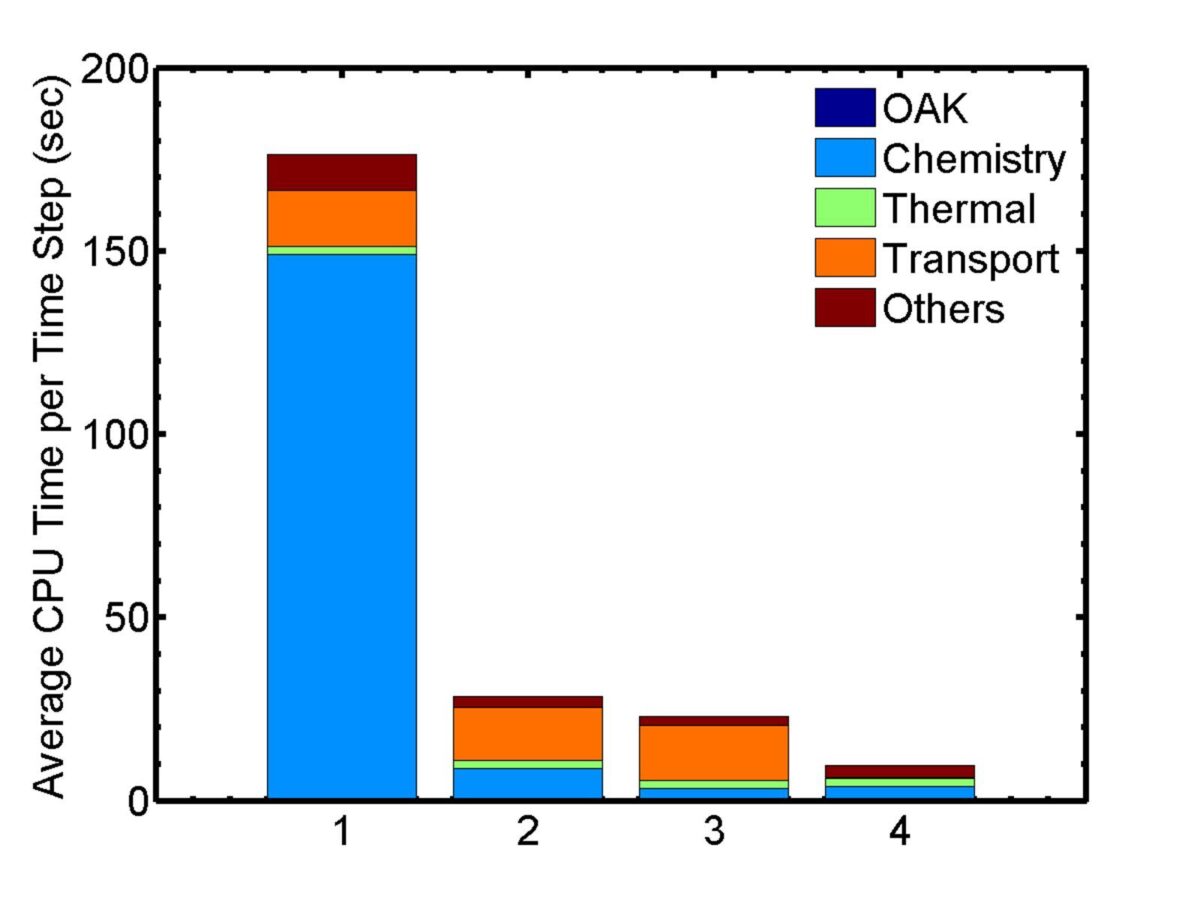
Average CPU time distribution per time step (sec) for four methods (from left to right): DVODE, ODEPIM, ODEPIM+OAK, and ODEPIM+OAK+CoTran
(2) Ozone Assisted Combustion
Plasma/ozone-assisted combustion is a promising technique to improve engine performance, increase lean burn flame stability, reduce emissions, and enhance low temperature fuel oxidation and processing. Plasma/ozone-assisted combustion takes advantage of the dramatically different kinetics between plasma/ozone and combustion to enhance and control the combustion process. We work on using plasma generated species to enhance and control combustion process. One ongoing project is to study the effect of ozone addition on combustion.
One well known reaction pathway to enhance combustion by ozone addition through ozone decomposition (O3àO2+O). Different from conventional understanding in which ozone was known to enhance flame speeds owing to its unique capability to release atomic oxygen (O3àO+O2) at elevated temperature conditions, We also discovered that ozone (through ozonolysis reactions) can induce explosive reactions at extremely low temperature conditions (even at room temperature) in combustion systems with unsaturated hydrocarbons, such as ethylene. It is common sense that combustion only occurs at high temperature conditions and can only be initiated by ignitors producing a high temperature environment. However, by taking advantage of ozonolysis reactions,(e.g., C2H4+O3àCH2O+H2+CO2, typically studied in atmosphere chemistry community regarding ozone layer depletion and pollution) autoignition was demonstrated at room temperature conditions. A new autoignition-assisted flame stabilization mechanism was also reported by us. Our work on ozone-enhanced combustion bridges the study in the atmosphere chemistry community and in the combustion community. It will provide a solution to enable low-temperature combustion and combustion at near limiting conditions for the development of advanced engines. This research also has the potential to develop a new aerated fuel injection technique and a fuel coking removal technique, therefore changing the cycle efficiency.
In this experiment, ozone is doped into synthetic air and ethylene is used as fuel to create autoigniting environment in diffusion jet flame. the flame dynamics of autoigniting flame is investigated. Figure below shows high speed images right after the fuel jet was turned on. Ozonolysis reactions between O3 and C2H4 produce large amount of CH2O and release heat. The chemiluminescence measurement indicates the formation of a cloud of CH2O, then an auignition kernel occured inside the CH2O cloud. In such a environment, flame could propagate orders of magnitude faster than its corresponding laminar flame speed.
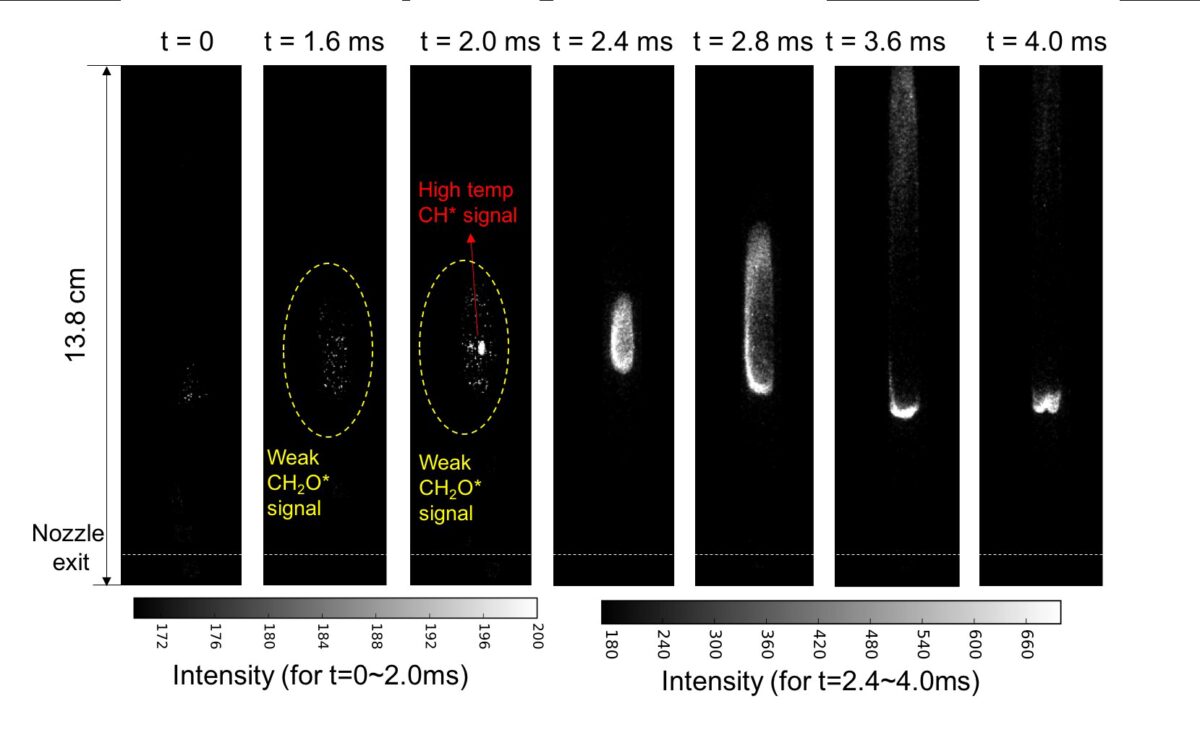
(3) High Pressure Combustion Kinetics
Recently, we developed a new and unique high pressure shock tube to study high pressure combustion kinetics. It enables measurement of critical fundamental combustion parameters in a completely new pressure region, especially those associated with combustion at a supercritical carbon dioxide (sCO2) condition suitable for a future power generation system. This is a regime where combustion kinetics has never been explored before. The sCO2 power cycle has higher efficiency and allows almost 100% carbon capture with no NOx emission (Zero Carbon Natural Gas, selected as 10 breakthroughs technologies in 2018 by MIT Technology Review). Once successful, this technique will potentially change the landscape of ground power generation. However, the sCO2 power cycle requires the combustor to run in the pressure range of 100 atm to 300 atm with high CO2 concentration, which is completely different from the operating regime of conventional gas turbines. For the first time in this field, we obtained autoignition delay of CH4/O2/CO2 autoignition delays at 100±7 atm at the sCO2 condition as shown in the plot below. Comparison with selected kinetic models shows that GRI 3.0 which is widely used in industries has been proven to have large deviation from experiments at sCO2 condition.
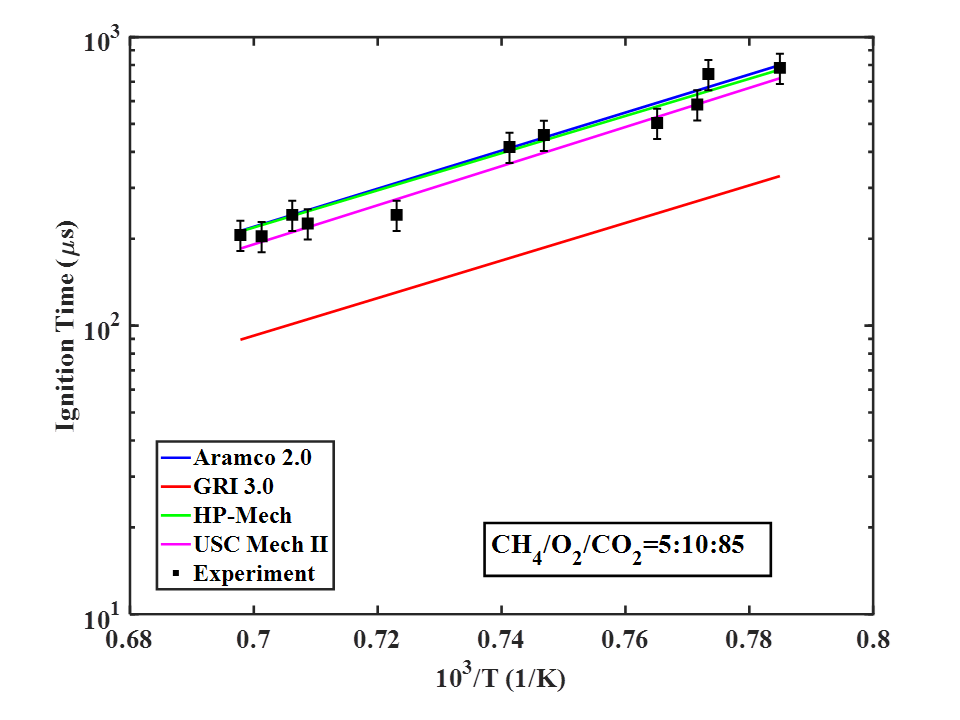
(4) Combustion Instability Control Using Plasma
In this project, we use non-equilibrium nanosecond pulsed plasma to control combustion instability. At a condition close to lean blowoff, flame starts to oscillate (a) and finally blows off with further decrease of equivalence ratio. With plasma activation, the lean blowoff limits can be significantly extended and flame can be stablized without osscilation (a'). At certain conditions, plasma also change the morphology of flames (b) and (b').
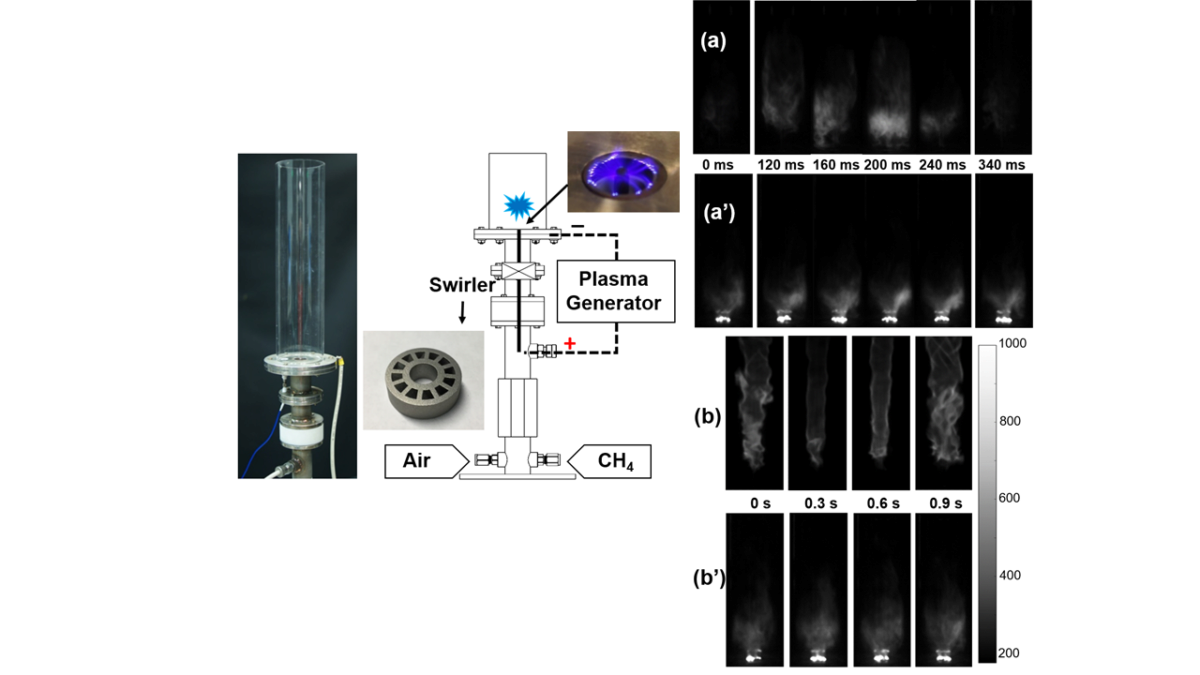
Bernard Lewis Fellowship, the Combustion Institute, 2012
Distinguished Paper, the 33rd International Symposium on Combustion, 2011
Selected Publications
[1]. W. Sun, Y. Ju, “Non-equilibrium plasma-assisted combustion: A review of recent progress” 2013 Journal of Plasma and Fusion Research, 89(4), 209-219 (invited paper)
[2]. B. Brumfield, W. Sun, Y. Ju, G. Wysocki “Detection of HO2 by Faraday rotation spectroscopy” 2013 J. Phys. Chem. Lett. 4(6), 872-876
[3]. W. Sun, S. H. Won, T. Ombrello, C. Carter, Y. Ju, “Direct ignition and the S-curve transition by in situ nano-second pulsed discharge in methane/oxygen/helium counterflow flame” 2013 Proceedings of the Combustion Institute, 34, 847-855
[4]. H. Guo, W. Sun, F. M. Haas, T. Farouk, F. Dryer, Y. Ju, “Measurements of H2O2 in low temperature dimethyl ether oxidation” 2013 Proceedings of the Combustion Institute, 34, 573-581
[5]. W. Sun, M. Uddi, S. H. Won, T. Ombrello, C. Carter, Y. Ju, “Kinetic effects of non-equilibrium plasma-assisted methane oxidization on diffusion flame extinction limits” 2012 Combustion and Flame, 159(1) 221-229
[6]. W. Sun, M. Uddi, T. Ombrello, S. H. Won, C. Carter, Y. Ju, “Effects of non-equilibrium plasma discharge on counterflow diffusion flame extinction” 2011 Proceedings of the Combustion Institute, 33, 3211-3218
[7]. W. Sun, Z. Chen, X. Gou, Y. Ju, “A path flux analysis method for the reduction of detailed chemical kinetic mechanisms” 2010 Combustion and Flame, 157(7) 1298-1307
[8]. W. Sun, G. Li, H. B. Wang, H. P. Li, C. Y. Bao, S. Zeng, “Characteristics of atmospheric-pressure, radio-frequency glow discharges operated with argon added ethanol” 2007 Journal of Applied Physics 101(12) 123302
[9]. W. Sun, T. R. Liang, H. B. Wang, H. P. Li, C. Y. Bao, “The back diffusion effect of air on the discharge characteristics of atmospheric-pressure radio-frequency glow discharges using bare metal electrodes” 2007 Plasma Sources Science and Technology 16 290-296